Scientists Debate Signatures of Alien Life
Searching for signs of life on faraway planets, astrobiologists must decide which telltale biosignature gases to target.
Huddled in a coffee shop one drizzly Seattle morning six years ago, the astrobiologist Shawn Domagal-Goldman stared blankly at his laptop screen, paralyzed. He had been running a simulation of an evolving planet, when suddenly oxygen started accumulating in the virtual planet’s atmosphere. Up the concentration ticked, from 0 to 5 to 10 percent.
“Is something wrong?” his wife asked.
“Yeah.”
The rise of oxygen was bad news for the search for extraterrestrial life.
After millennia of wondering whether we’re alone in the universe — one of “mankind’s most profound and probably earliest questions beyond, ‘What are you going to have for dinner?’” as the NASA astrobiologist Lynn Rothschild put it — the hunt for life on other planets is now ramping up in a serious way. Thousands of exoplanets, or planets orbiting stars other than the sun, have been discovered in the past decade. Among them are potential super-Earths, sub-Neptunes, hot Jupiters and worlds such as Kepler-452b, a possibly rocky, watery “Earth cousin” located 1,400 light-years from here. Starting in 2018 with the expected launch of NASA’s James Webb Space Telescope, astronomers will be able to peer across the light-years and scope out the atmospheres of the most promising exoplanets. They will look for the presence of “biosignature gases,” vapors that could only be produced by alien life.
They’ll do this by observing the thin ring of starlight around an exoplanet while it is positioned in front of its parent star. Gases in the exoplanet’s atmosphere will absorb certain frequencies of the starlight, leaving telltale dips in the spectrum.
Filming by Tom Hurwitz and Richard Fleming. Editing and motion graphics by Ryan Griffin. Other graphics and images from NASA, the European Southern Observatory and Creative Commons. Music by Podington Bear.
In Theory Video: David Kaplan explores the best ways to search for alien life on distant planets.
As Domagal-Goldman, then a researcher at the University of Washington’s Virtual Planetary Laboratory (VPL), well knew, the gold standard in biosignature gases is oxygen. Not only is oxygen produced in abundance by Earth’s flora — and thus, possibly, other planets’ — but 50 years of conventional wisdom held that it could not be produced at detectable levels by geology or photochemistry alone, making it a forgery-proof signature of life. Oxygen filled the sky on Domagal-Goldman’s simulated world, however, not as a result of biological activity there, but because extreme solar radiation was stripping oxygen atoms off carbon dioxide molecules in the air faster than they could recombine. This biosignature could be forged after all.
The search for biosignature gases around faraway exoplanets “is an inherently messy problem,” said Victoria Meadows, an Australian powerhouse who heads VPL. In the years since Domagal-Goldman’s discovery, Meadows has charged her team of 75 with identifying the major “oxygen false positives” that can arise on exoplanets, as well as ways to distinguish these false alarms from true oxygenic signs of biological activity. Meadows still thinks oxygen is the best biosignature gas. But, she said, “if I’m going to look for this, I want to make sure that when I see it, I know what I’m seeing.”
Meanwhile, Sara Seager, a dogged hunter of “twin Earths” at the Massachusetts Institute of Technology who is widely credited with inventing the spectral technique for analyzing exoplanet atmospheres, is pushing research on biosignature gases in a different direction. Seager acknowledges that oxygen is promising, but she urges the astrobiology community to be less terra-centric in its view of how alien life might operate — to think beyond Earth’s geochemistry and the particular air we breathe. “My view is that we do not want to leave a single stone unturned; we need to consider everything,” she said.
As future telescopes widen the survey of Earth-like worlds, it’s only a matter of time before a potential biosignature gas is detected in a faraway sky. It will look like the discovery of all time: evidence that we are not alone. But how will we know for sure?
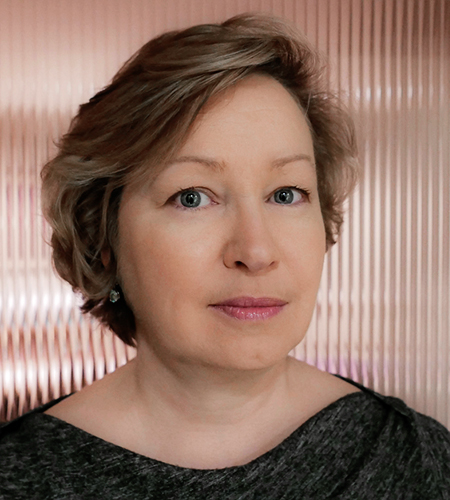
Courtesy of Victoria Meadows
Victoria Meadows, an astrobiologist and principal investigator of the University of Washington’s Virtual Planetary Laboratory.
Scientists must quickly hone their models and address the caveats if they are to select the best exoplanets to target with the James Webb telescope. Because of the hundreds of hours it will take to examine the spectrum for each planetary atmosphere and the many competing demands on its time, the telescope will likely only observe between one and three earthlike worlds in the habitable “Goldilocks” zones of nearby stars. In choosing from a growing list of known exoplanets, the scientists want to avoid planetary circumstances in which oxygen false positives arise. “We’re looking at maybe putting our eggs, if not all in one basket, at least in only a couple of baskets,” Meadows said, “so it’s important to try and figure out what we should be looking for there. And in particular, how we might get fooled.”
Breath of Life
Oxygen has been regarded as the gold standard since the chemist James Lovelock first contemplated biosignature gases in 1965, while working for NASA on methods of detecting life on Mars. As Frank Drake and other pioneers of astrobiology sought to detect radio signals coming from distant alien civilizations — an ongoing effort called the search for extraterrestrial intelligence (SETI) — Lovelock reasoned that the presence of life on other planets could be deduced by looking for incompatible gases in their atmospheres. If two gases that react with each other can both be detected, then some lively biochemistry must be continually replenishing the planet’s atmospheric supplies.
In Earth’s case, though it readily reacts with hydrocarbons and minerals in the air and ground to produce water and carbon dioxide, diatomic oxygen (O2) comprises a steady 21 percent of the atmosphere. Oxygen persists because it is poured into the sky by Earth’s photosynthesizers — plants, algae and cyanobacteria. They enlist sunlight to strip hydrogen atoms off water molecules, building carbohydrates and releasing the oxygen byproduct as waste. If photosynthesis ceased, the existing oxygen in the sky would react with elements in the crust and drop to trace levels in 10 million years. Eventually, Earth would resemble Mars, with its carbon dioxide-filled air and rusty, oxidized surface — evidence, Lovelock argued, that the Red Planet does not currently harbor life.
But while oxygen is a trademark of life on Earth, why should that be true elsewhere? Meadows argues that photosynthesis offers such a clear evolutionary advantage that it is likely to become widespread in any biosphere. Photosynthesis puts the biggest source of energy on any planet, its sun, to work on the most commonplace of planetary raw materials: water and carbon dioxide. “If you want to have the uber-metabolism you will try and evolve something that will allow you to use sunlight, because that’s where it’s at,” Meadows said.
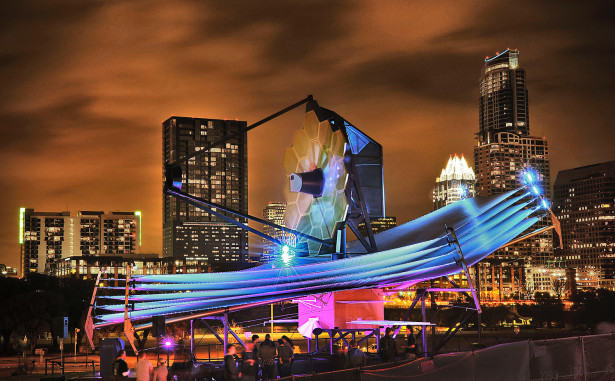
NASA/Chris Gunn
A full-scale model of the tennis court-size James Webb Space Telescope on display in Austin, Tex., in 2013.
Diatomic oxygen also boasts strong absorption bands in the visible and near-infrared — the exact sensitivity range of both the $8 billion James Webb telescope and the Wide Field Infrared Survey Telescope (WFIRST), a mission planned for the 2020s. With so many imminent hopes riding on oxygen, Meadows is determined to know “where the gotchas are likely to be.” So far, her team has identified three major nonbiological mechanisms that can flood an atmosphere with oxygen, producing false positives for life. On planets that formed around small, young M-dwarf stars, for instance, intense ultraviolet sunlight can in certain cases boil down the planet’s oceans, creating an atmosphere thick with water vapor. At high altitudes, as VPL scientists reported in the journal Astrobiology last year, intense UV radiation splinters off the lightweight hydrogen atoms. These atoms then escape to space, leaving behind a veil of oxygen thousands of times denser than Earth’s atmosphere.
Because the smallness of M-dwarf stars makes it easier to detect much smaller, rocky planets passing in front of them, they are the intended targets for NASA’s Transiting Exoplanet Survey Satellite (TESS), a planet-finding mission scheduled to launch next year. The earthlike planets that will be studied by the James Webb telescope will be selected from among TESS’s finds. With these candidates on the way, astrobiologists must learn how to distinguish between alien photosynthesizers and runaway ocean boiling. In work that is now being prepared for publication, Meadows and her team show that a spectral absorption band from tetraoxygen (O4) loosely forms when O2molecules collide. The denser the O2 in an atmosphere, the more molecular collisions occur and the stronger the tetraoxygen signal becomes. “We can look for the [O4] to give us the telltale sign that we’re not just looking at a 1-bar atmosphere with 20 percent oxygen” — an earthlike atmosphere suggestive of photosynthesis — Meadows explained, “we’re looking at something that just has massive amounts of oxygen in it.”
A strong carbon monoxide signal will identify the false positive that Domagal-Goldman first encountered that drizzly morning in 2010. Now a research scientist at NASA’s Goddard Space Flight Center in Greenbelt, Md., he says he isn’t worried about oxygen’s long-term prospects as a reliable biosignature gas. Oxygen false positives only happen in rare cases, he said, “and the planet that has those certain cases is also going to have observational properties that we should be able to detect, as long as we think about it in advance, which is what we’re doing right now.”
He and other astrobiologists are also mindful, though, of oxygen false negatives — planets that harbor life but have no detectable oxygen in their atmospheres. Both the false positives and false negatives have helped convince Sara Seager of the need to think beyond oxygen and explore quirkier biosignatures.
Encyclopedia of Gases
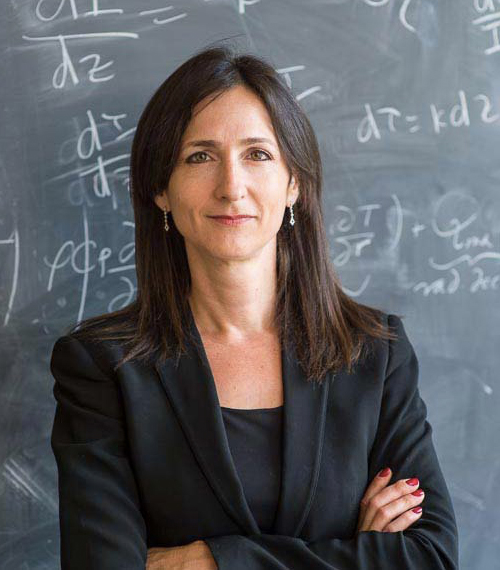
Courtesy of Sara Seager
Sara Seager, an astrophysicist and planetary scientist at the Massachusetts Institute of Technology.
If the diverse exoplanet discoveries of the past decade have taught us anything, it’s that planetary sizes, compositions and chemistries vary dramatically. By treating oxygen as the be-all, end-all biosignature gas, Seager argues, we might miss something. And with a personal dream of discovering signs of alien life, the 44-year-old can’t abide by that.
Even on Earth, Seager points out, photosynthesizers were pumping out oxygen for hundreds of millions of years before the process overwhelmed Earth’s oxygen sinks and oxygen started accumulating in the sky, 2.4 billion years ago. Until about 600 million years ago, judged from a distance by its oxygen levels alone, Earth might have appeared lifeless.
Meadows and her collaborators have studied some alternatives to oxygenic photosynthesis. But Seager, along with William Bains and Janusz Petkowski,are championing what they call the “all-molecules” approach. They’recompiling an exhaustive database of molecules — 14,000 so far — that could plausibly exist in gas form. On Earth, many of these molecules are emitted in trace amounts by exotic creatures huddled in ocean vents and other extreme milieus; they don’t accumulate in the atmosphere. The gases might accrue in other planetary contexts, however. On methane-rich planets, as the researchers argued in 2014, photosynthesizers might harvest carbon from methane (CH4) rather than CO2 and spew hydrogen rather than oxygen, leading to an abundance of ammonia. “The ultimate, long-term goal is [to] look at another world and make some informed guesses as to what life might produce on that world,” said Bains, who splits his time between MIT and Rufus Scientific in the United Kingdom.
Domagal-Goldman agrees that thinking both deeply about oxygen and broadly about all the other biochemical possibilities is important. “Because all these surprises have happened in our detections of the masses and radii and orbital properties of these other worlds,” he said, “[astronomers] are going to keep pushing on the people like me who come from an earth sciences background, saying, ‘Let’s think further outside the box.’ That is a healthy and necessary pressure.”
Meadows, however, questions the practicality of the all-molecules approach. In a 3,000-word email critiquing Seager’s ideas, she wrote, “After you build this exhaustive database, how do you identify those molecules that are most likely to be produced by life? And how do you identify their false positives?” She concluded: “You will still have to be guided by life on Earth, and our understanding of planetary environments and how life interacts with those environments.”
In contemplating what life might be like, it’s exasperatingly difficult to escape the only data point we have — for now.
Uncertain Odds
At a 2013 symposium, Seager presented a revised version of the Drake equation, Frank Drake’s famous 1961 formula for gauging the odds that SETI would succeed. Whereas the Drake equation multiplied a string of mostly unknown factors to estimate the number of radio-broadcasting civilizations in the galaxy, Seager’s equation estimates the number of planets with detectable biosignature gases. With the modern capacity to look for any life regardless of whether it’s intellectually capable of beaming messages into space, the calculation of our chances of success no longer depends on uncertainties like the rareness of intelligence as an evolutionary outcome or the galactic popularity of radio technology. However, one of the biggest unknowns remains: the probability that life will arise in the first place on a rocky, watery, atmospheric planet like ours.
“Abiogenesis,” as the mystery event is called, seems to have occurred not long after Earth accumulated liquid water, leading some to speculate that life might start up readily, even inevitably, under favorable conditions. But if so, then shouldn’t abiogenesis have happened multiple times in Earth’s 4.5-billion-year history, spawning several biochemically distinct lineages rather than a monoculture of DNA-based life?John Baross, a microbiologist at the University of Washington who studies the origins of life, explained that abiogenesis might well have happened repeatedly, creating a menagerie of genetic codes, structures and metabolisms on early Earth. But gene-swapping and Darwinian selection would have merged these different upstarts into a single lineage, which has since colonized virtually every environment on Earth, preventing new upstarts from gaining ground. In short, it’s virtually impossible to tell whether abiogenesis was a fluke event, or a common occurrence — here, or elsewhere in the universe.
Scheduled to speak last at the symposium, Seager set a light-hearted tone for the after party. “I put it all in our favor,” she said, positing that life has a 100 percent chance of arising on Earth-like planets, and that half of these biospheres will produce detectable biosignature gases — another uncertainty in her equation. Crunching these wildly optimistic numbers yielded the prediction that two signs of alien life would be found in the next decade. “You’re supposed to laugh,” Seager said.
Meadows, Seager and their colleagues agree that the odds of such a detection this decade are slim. Though the prospects will improve with future missions, the James Webb telescope would have to get extremely lucky to pick a winner in its early attempts. And even if one of its targeted planets does harbor life, spectral measurements are easily foiled. In 2013, the Hubble Space Telescope monitored the starlight passing through the atmosphere of a midsized planet called GJ 1214b, but the spectrum was flat, with no chemical fingerprints at all. Seager and her collaboratorsreported in Nature that a high-altitude layer of clouds appeared to have obscured the planet’s sky from view.
SOURCE.... QUANTA MAGAZINE